Join the others who are receiving high-signal, data-driven analysis to be in front of their peers in the cannabinoid space! If you have found value in our insights please share this with another canna-curious individual to grow the revolution!
Please note this is part of our Monthly Playbook. If you would like to read the entire Monthly Playbook you can sign up here. The library can be found here
In the world of manufacturing, things move quickly. The manufacturer’s goal is to manufacture their product with the target quality in the proper quantity with as little waste possible. Many manufacturing outlets will do their quality control before a process starts and after the process ends, but what about while something is being created?
Welcome to The ABCs of cannabinoid manufacturing
Part 5: Extraction Methods
The separation of the wide array of compounds in Cannabis sativa is becoming more important as regulations on cannabinoid containing products become more stringent. There are a few common methodologies used for extraction in the cannabis industry.
Depending on the intended final product, incoming processing can include separation of the flower portions with the highest concentration of cannabinoids and terpenes. Then the extraction of the terpenes and the cannabinoids can begin. Though mechanical separation methods exist, this article will focus on the most common solvent-based extractions: hydrocarbon, ethanol and super-critical CO2 . Solvent extraction methodologies have been around for millennia since the ancient Egyptians made tinctures by soaking herbs in ethanol. Tinctures are created by putting the herbs into a liquid, usually alcohol, to extract the flavors, healing components, etc.
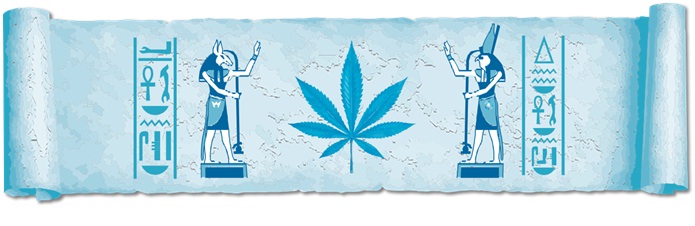
Basic Solvent Extraction
The basic solvent extraction is based on historical methods, although we have made technological advances to help us control the processes over the centuries. Today, common organic solvents include ethanol [1], butane, and propane. [2] Water extraction will not be covered in this article due to its low yields of cannabinoids and terpenes.
Alcohol extraction uses ethanol and is one of the most common and efficient methods of extracting the cannabinoids. The initial step is to soak the raw material in Ethanol to remove the trichomes. The polar end (-OH group) of the molecule helps dissolve the hydrophilic compounds, such as chlorophyll. The non-polar end (C2¬H5) helps to dissolve the hydrophobic compounds such as the plant waxes, oils, cannabinoids, and terpenes.
The process can be done in either warm or cold ethanol. In warm or room temperature ethanol, the cannabinoids dissolve quickly leading to a high yield of cannabinoids. However, the warm ethanol extraction process also dissolves plant lipids including the chlorophyll, which causes a strong bitter taste. By dropping the temperature of the ethanol to less than -30˚C (-22˚F), you decrease the solubility of all the compounds leading to a much slower dissolution of the products into the ethanol. However, it is also below the freezing point of many plant waxes which means that many of the compounds will be solids. The impurities separate from the cannabinoids and can be easily removed.
Hydrocarbon extraction
Hydrocarbon extraction generally uses either propane or butane. Propane and butane are small hydrocarbons, made only of carbon and hydrogen atoms, that are non-polar with low boiling points, (-44˚F and 32˚F, respectively) [3]. The initial extraction washes the raw material with the cold hydrocarbon.
The non-polarity of the molecules helps easily dissolve the cannabinoids, waxes, fats and lipids. Unlike ethanol, there is no polar end to help with the dissolution of certain undesired compounds such as chlorophyll. The terpenes are also easily dissolved in the hydrocarbons, though the flavonoids have limited solubility. The hydrocarbons with the products of interest can be separated by flowing the mixture into a separate area and raising the temperature. [4] With low boiling points, the hydrocarbons evaporate at -44˚F (Propane) and 32˚F (Butane) leaving behind the waxes, fats, lipids, cannabinoids, and terpenes.
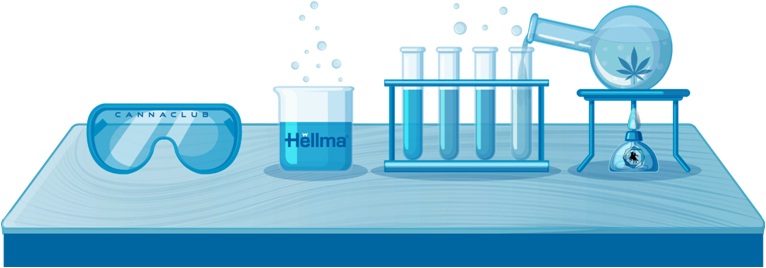
Final extraction method
After the separation of the hydrocarbons from the extract, the propane or butane can be recirculated though the biomass creating a closed-looped system. The result is an extract that is relatively free of inactive plant matter such as chlorophyll. Hydrocarbon extraction is losing popularity primarily due to regulations for handling propane/butane and stigma attached to a using a hazardous chemical for the extraction.
The final extraction method uses super-critical CO2 . Outside the cannabinoid industry, supercritical extraction methods are used for the production of high-quality hempseed oil, extracting caffeine from coffee, removing pesticides from agriproducts, etc. [5].
At standard temperatures and pressures (room temperature and sea-level atmospheric pressure), all molecules are in their natural state of matter: solid, liquid, gas, or plasma. One can change the state of matter by changing the temperature or the pressure or both. A good example of this would be creating ice cubes in your freezer. Without changing the pressure, you can turn the water into ice by lowering the temperature.
When modulating the temperature and pressure of a system, liquids and gases can hit a critical point where they exhibit characteristics of both liquid and gas. They take up the entire space (more compressible) like gases and have liquid-like densities. This is called a supercritical fluid. [7]. Figure 2 shows the phase diagram of Carbon Dioxide (CO2 ). Using CO2 has several advantages, it is nonflammable, non-toxic, relatively inert, abundant and inexpensive [8, 9].
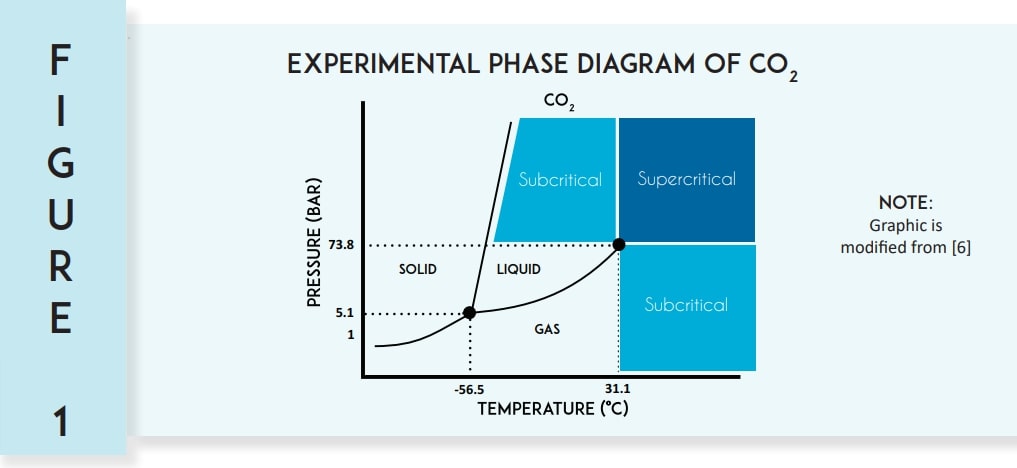
The other main advantage is that at a temperature of 31˚C, you can maintain the supercritical liquid at 74 bar [6, 7]. The various components of cannabis have different solubilities at different temperatures and pressures, thus allowing a clean extraction of the target compounds. However, one study has shown that the concentrations of different products can be extracted at different rates, so the extract should be analyzed [2, 8, 10].
MISSED PART FOUR?
Expanded versions of previous article installments are available upon request.
Hellma USA, Inc has been serving the U.S. markets in spectroscopic accessories for over 50 years. They specialize in quartz manufacturing for the lab and probes for the process. Dr. Carrier earned her Ph.D. from University of Wisconsin – Madison in Physical Chemistry specializing in Spectroscopic techniques to monitor chemical reactions